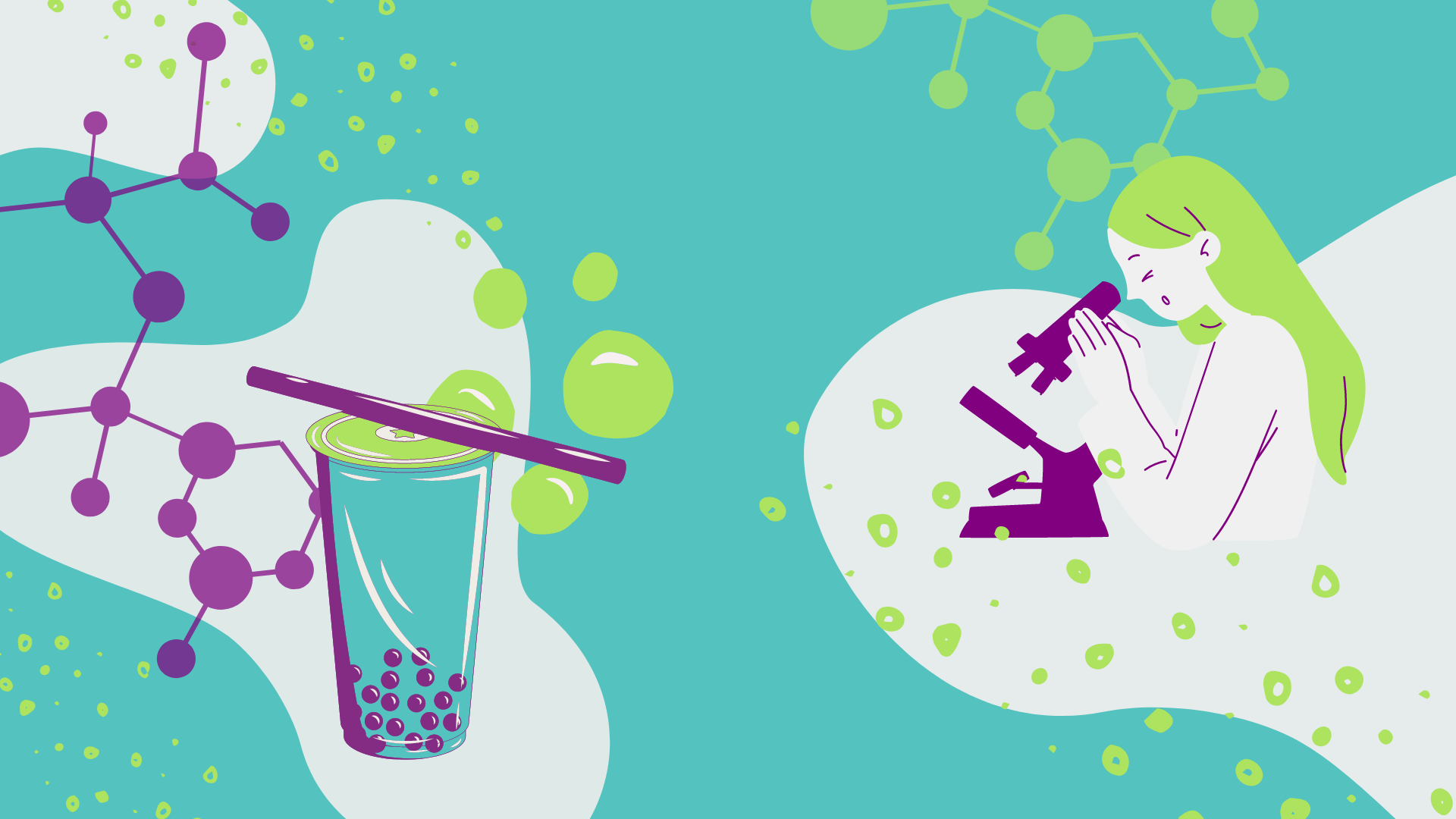
Hydrogels: from Bubble Tea to Cancer Therapy
Shiqi Wang is a postdoctoral researcher at the Faculty of Pharmacy, University of Helsinki. Her research focuses on developing hydrogels and polymer materials for drug delivery.
This article is part of the intersections theme.
edited by Kenia, reviewed by carmine d’amico, illustrated by sophie hoetzel, read by emmi olkkonen.
Bubble tea – a drink that originated in Taiwan but is beloved globally by countless fans. Its unique combination of tea flavours and chewy, mouth-popping pearls has made it a favourite among beverage enthusiasts everywhere. Yet, few ever wonder about the science behind those tiny orbs. How can they “magically” seal juice within? And how are they connected with contact lenses, smart drug delivery systems, and live cells being used to treat incurable diseases?
The magic of bubble tea
Bubble tea has many different flavours and toppings, but for this article’s purposes, let me just introduce two types of chewy balls: black “pearls”, commonly added in traditional pearl tea, and “popping boba”, flavoured spheres with a thin, gel-like skin and filled with juice inside. Both are what we call hydrogels.
Hydrogels, a term that contains two words, “hydro” for water and “gel” signifying a state between liquid and solid, are known for their capacity to absorb and retain vast amounts of water. Thanks to a 3D network structure, hydrogels can hold the liquid and maintain their shape even when their composition is up to 80% water (as you will soon see!). Although this 3D structure is always similar at a molecular level, hydrogels can be quite different in terms of shape, softness, and texture depending on the key component that keeps the water in. In the case of black “pearls”, the chewy ball is made of natural starch from tapioca roots via hydrogen bonds, a type of chemical interaction between a positively charged hydrogen atom and a negatively charged one such as oxygen. As for “popping boba”, the key component is alginate, a negatively charged compound commonly found in seaweed, and linked by positively charged calcium ions. As common wisdom goes, opposites attract, and the network sticks together!
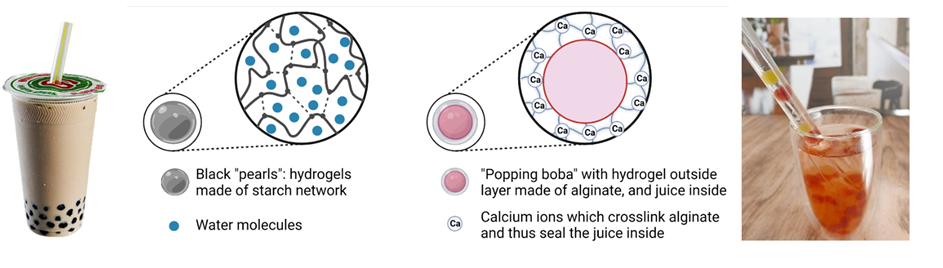
Hydrogels: from bubble tea to smart drug delivery
Besides eating them in your bubble tea, you encounter hydrogels more often than you think. They are favoured by various industries and fields because it is possible to adjust their mechanical properties, such as flexibility and hardness, by manipulating the density of linkages and adjusting the materials of their 3D network. For example, we can find hydrogels in various cosmetics, such as moisturizers, serums and face masks. In these products, hydrogels are usually soft and spreadable, not holding a defined shape. But we can also find them as contact lenses and breast implants, where they are tougher, but still stretchable, thanks to a “denser” network with more links and a different matrix (see Figure 2).
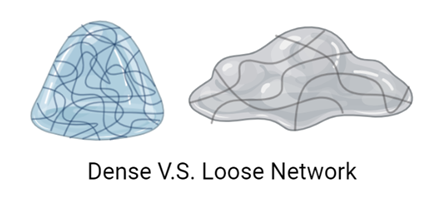
This versatility also makes hydrogels a commonly used molecule in smart drug delivery systems. As you might imagine from their name, these systems can deliver drugs to specific diseased sites, which improves therapeutic efficacy. By adjusting the hydrogel properties and making them similar to the corresponding tissue we want to apply it to, we can increase their compatibility with our body. For example, ALGICELL® Ag is a hydrogel used to treat from minor cuts to chronic wounds such as difficult-to-heal diabetic ulcers. Since its purpose is to be an antimicrobial wound-dressing, it has been adjusted to have a similar toughness and stretchability as our skin. This creates a “skin-mimicking hydrogel layer” that acts as a barrier to prevent further infections while helping the wound close faster. And guess what? The major components of ALGICELL® Ag are the same as in “popping boba” but with a small amount of silver ions added as “active drugs” to inhibit bacteria growth in the wounded area.
Another advantage of using hydrogels for drug delivery is that their 3D network structure (remember, this is the hydrogel matrix keeping the liquid in) can temporarily break but quickly re-organize once the breaking force is removed. This so-called “self-healing” property, known as dynamic network, enables us to inject the hydrogels through syringes and needles. After the injection, with the removal of the force (the penetrating needle), the hydrogels will recover, remaining intact instead of breaking into smaller pieces.
In an extreme (but fun) case, scientists have developed super jelly hydrogels which can withhold the weight of a car and recover its original shape despite their 80 percent water content:
The self-healing property is also very useful for precise control of drugs in the body. For example, smart hydrogels have been developed using alginate and calcium as a matrix (yes, the same as popping boba again!) that release drugs only when triggered by “signals”. When an ultrasound is applied (the “signal”), the hydrogel network temporarily breaks at molecular scale, opening up and releasing the encapsulated drugs. When the ultrasound stops, the network swiftly re-arranges itself and withholds the rest of the drugs. The hydrogels are thus like a drug reservoir inside the body, responding to non-invasive ultrasound triggers from the outside.
In cancer chemotherapy on animals, for example, results have shown that cancer cells are more likely to get killed when they are hit by the bursts of high-dose chemotherapy drugs released by hydrogels, compared with the same dose delivered through conventional therapies, where drug concentration stays low. This translates into better therapeutic efficacy, since the chemotherapy drugs can be given in a more precise way, in the quantities they are needed, to maximize their anti-cancer effects and reduce the side effects. But hydrogels’ potential for delivering treatment goes even further, as they can carry much more than just traditional drug molecules. In fact, scientists have recently been using them to deliver not only proteins but even live cells to treat previously incurable diseases.
Live cells and new perspectives in tissue regeneration
Live cells show huge potential as a game-changer in the regenerative biomedicine field because some organs like the heart and the brain have limited capability to regenerate. Thus, damage on heart tissues and neurons after a heart attack or a stroke can be permanent, causing function loss and long-term effects on the patients’ life. But what if we could re-introduce new healthy cells in the damaged region? Would these organs be able to use them to heal themselves and reverse the effects of the disease?
Theoretically, yes! But in reality, it is super challenging. That is why pairing live cells with hydrogels bears so much potential. Live cells, unlike traditional drug molecules, have complicated structures and require exquisite conditions for survival and growth, including comfortable physical supports and nutrients to stimulate their growth. By encapsulating living cells together with their required nutrients, hydrogels meet these conditions and act as a tiny “3D cell incubator” around the damaged organ. For example, scientists have developed hydrogels carrying live stem cells, cells capable of growing into different organs, for heart attack treatment. The results? Better recovery and less scarring on the heart.
Where science intersects with everyday life
During the years I have studied the potential of hydrogels as smart drug delivery systems in cancer therapy, I have gradually realized that these injectable soft and jelly materials are little more than an obtuse scientific term to most. But hydrogels, albeit virtually unknown to the general public, withhold great potential to deliver all kinds of medicines and even live cells.
My hope is that next time you take a sip of bubble tea, you are reminded of how cutting-edge science and research can intersect with everyday life, and wonder about how even a small chewy ball can hold within the potential to save millions of lives in the future.
references
Guedes, G., Wang, S., Fontana, F., Figueiredo, P., Lindén, J., Correia, A., … Santos, H. A. (2021). Dual‐Crosslinked Dynamic Hydrogel Incorporating {Mo 154 } with pH and NIR Responsiveness for Chemo‐Photothermal Therapy. Advanced Materials, 33(40), 2007761. https://doi.org/10.1002/adma.202007761
Hastings, C. L., Roche, E. T., Ruiz-Hernandez, E., Schenke-Layland, K., Walsh, C. J., & Duffy, G. P. (2015). Drug and cell delivery for cardiac regeneration. Advanced Drug Delivery Reviews, 84, 85–106. https://doi.org/10.1016/j.addr.2014.08.006
Huang, Z., Chen, X., O’Neill, S. J. K., Wu, G., Whitaker, D. J., Li, J., … Scherman, O. A. (2022). Highly compressible glass-like supramolecular polymer networks. Nature Materials, 21(1), 103–109. https://doi.org/10.1038/s41563-021-01124-x
Huang Zhuojun. (2018). Breaking Boba – the chemistry of bubble tea. Retrieved November 28, 2022, from https://itsamaterialsworld.wordpress.com/2018/12/26/breaking-boba-the-chemistry-of-bubble-tea-pt-1/
Huebsch, N., Kearney, C. J., Zhao, X., Kim, J., Cezar, C. A., Suo, Z., & Mooney, D. J. (2014). Ultrasound-triggered disruption and self-healing of reversibly cross-linked hydrogels for drug delivery and enhanced chemotherapy. Proceedings of the National Academy of Sciences, 111(27), 9762–9767. https://doi.org/10.1073/pnas.1405469111
Li, J., & Mooney, D. J. (2016). Designing hydrogels for controlled drug delivery. Nature Reviews Materials, 1(12), 16071. https://doi.org/10.1038/natrevmats.2016.71
Mandal, A., Clegg, J. R., Anselmo, A. C., & Mitragotri, S. (2020). Hydrogels in the clinic. Bioengineering & Translational Medicine, 5(2). https://doi.org/10.1002/btm2.10158
Notes about Figures and Video used in this blog:
The pictures of the Bubble tea (first figure in the article) are from Wikipedia under CC BY-SA 3.0 and CC BY-SA 4.0 licence. Original files: https://en.wikipedia.org/wiki/Bubble_tea#/media/File:Bubble_Tea.png https://en.wikipedia.org/wiki/Popping_boba#/media/File:Popping_boba_w_Bubble_Tea.jpg,.