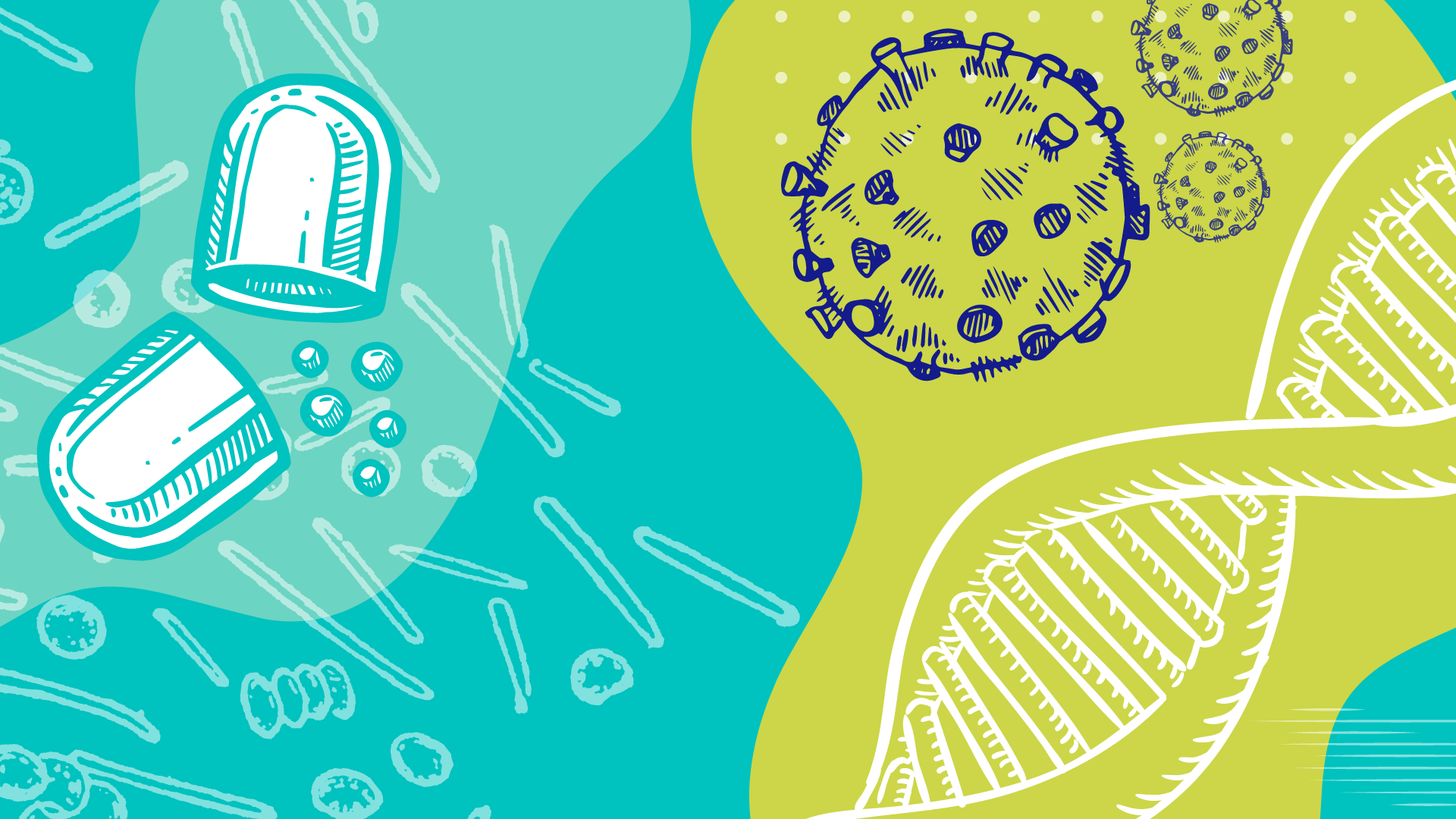
Is the world heading back to a pre-antibiotic era?
Maritta Keurulainen is a PhD researcher at university of helsiki studying bacterial communication (quorum sensing). Her research focuses on trying to reduce pathogenicity, restoring functionality of antibiotics and finding new antimicrobials for E. COLI CAUSING URINARY TRACT INFECTIONS.
This article is part of the innovation theme.
edited by Kenia, reviewed by vinaya dulipati, and illustrated by jelena matovic.
As patients, doctors often remind us how important it is to complete prescribed antibiotic treatments to prevent the development of antibiotic resistance by the infecting microbes. But how many of us ever consider that not following these instructions may mean that, in the future, surgeries will become impossible? How many have ever given a thought to the idea that common infectious diseases, such as pneumonia, urinary tract infections and sepsis, could – once again – become deadly?
You might think that I’m describing a futuristic scenario. Unfortunately, you would be wrong. Antimicrobial resistance (AMR) is sometimes referred to as “the silent pandemic”, its numbers are growing faster than expected, but the issue remains mostly under the radar for the general public. A study conducted in the UK in 2014 estimated that if AMR is left unaddressed, multidrug-resistant (MDR) bacterial infections would be responsible for 10 million deaths yearly on a global scale before 2050. That is more than the predicted cancer deaths during the same period. In 2014, infectious diseases not treatable with antibiotics (that is, infections caused by antibiotic-resistant microbes) had already killed approximately 700,000 people worldwide. In 2019, the yearly death toll had risen to almost 1.3 million.
What has led us to this grim situation? Bacteria develop resistance, first and foremost, because it’s a question of life or death for them to do so. Resistance equals survival, as current antibiotics either kill bacteria or slow down their growth. A second reason for the situation is the unwarranted use of antibiotics by humans and farms, both land and aquatic, causing unnecessary selection pressure on bacteria to develop resistance. For example, most respiratory infections are caused by viruses, but doctors sometimes prescribe patients antibiotics without proper diagnostic tests to identify the causing microbe, even though they are ineffective against viral infections. On the other hand, antibiotics are often added to animal feed in agriculture for reasons other than to treat diseases. In some cases, it is to prevent them from getting sick due to the overcrowded conditions in industrial rearing. In others, common antibiotics, such as penicillin or tetracycline, are used in certain countries as growth enhancers due to their ability to make animals gain weight.
In both scenarios, antibiotics end up in the environment as excretions and expose free roaring bacteria to them. As a result, environmental bacteria develop resistance to antibiotics that ends up in disease-causing bacteria due to these microorganisms’ ability to share the resistance genes. The final outcome of this chain of events is antibiotic-resistant infections in humans and animals, which may lead to death or sepsis if alternative treatments aren’t available.
Another challenge is that pharmaceutical companies have not introduced new antibiotics in the last 30 years. The average antibiotic treatment for a patient lasts seven to ten days at a time. Therefore, compared to, for example, blood pressure or type-2 diabetic drugs, which are taken daily for decades, an antibiotic needs to be prescribed to many people to bring back the money that the pharmaceutical company invested in its development. The timeframe for profit is also becoming increasingly shorter, as resistance has started to evolve for every new existing antibiotic only a few years after its release to the market. Moreover, every new antibiotic brought to market is put in reserve by doctors and hospitals as the last resort treatment to avoid the development of resistance, which again brings no profit to the pharmaceutical company which developed it. In fact, in the last few years, several smaller companies focusing on the development of antibiotics have gone bankrupt because of this. Several drug candidates are currently undergoing clinical trials, but they are not expected to cure the antimicrobial problem as they mainly target the same functions as previous antibiotics. As the antibiotic resistance problem exacerbates, we need innovative new approaches to combat the challenge.
Unlike assumed in the past, bacteria are not lonely riders. They are social entities that communicate with each other and take action accordingly. This communication system, called quorum sensing, is relayed through small molecules called autoinducers. You can think of these molecules as leaves dropped in a spot in a lake. Over time, they drift further and further from their starting point and start accumulating in the environment in a density-dependent manner: the more bacterial cells, the more autoinducers. Additionally, as trees produce leaves of different colours and shapes, different bacteria also produce identifiable autoinducers that allow them to sense the size and composition of their community and whether those around them are friends or competitors.
When it comes to infections, the right timing is everything. When the bacteria know, from the number of autoinducers, that the community is large enough to overwhelm its host, they launch an attack with a higher chance of succeeding. Bacteria communicate with each other because launching an attack at the wrong moment would be a costly mistake and a waste of energy and materials. Low bacteria numbers during an attack could be efficiently eliminated by the host’s immune system, as a police force would do to a small group of unorganized troublemakers. Additionally, bacteria need to start producing what are known as virulence factors, an array of complex molecules, to prepare for the attack.
But what if this communication between bacteria could be interrupted? Could we develop “anti-messaging agents”, drugs/molecules that could block quorum sensing? In such a situation, the bacteria would send messages to each other, but the “letters” would be lost in the mail. Therefore, the bacteria would never realize that their army is large and strong enough to overwhelm the host, no attack would occur, and there would be no disease. Furthermore, as these “anti-messaging” agents would not kill the bacteria nor slow down their growth, the bacteria would have no reason to develop resistance against them.
Problem solved, might the reader think. Unfortunately, the reality is slightly more complicated than I just illustrated. Multiple issues must be addressed before any “anti-messaging” agents hit the market. The beneficial bacteria in our gut also communicate, and we do not want to harm them like antibiotics do. Additionally, different communication systems exist, and the same bacterium may utilize up to three different ones. Thus, even if we block one communication system, the bacteria can switch over to using another one. Moreover, these communication systems are connected in ways we still don’t fully understand.
Currently, multiple research groups and projects are tackling quorum sensing by focusing on specific disease-causing bacteria, such as Pseudomonas aeruginosa, responsible for cystic fibrosis, and Escherichia coli, one of the main causes of urinary tract infections. But what can you, the reader, do about this issue? You can stop using antibiotics whenever it is not absolutely necessary. You can lobby your authorities and foundations to invest in this field of research. You can press your authorities to pass laws to prevent using antibiotics as preventives or growth enhancers in agriculture. Small grass-root actions do matter, and they have far-reaching results. Every step in the right direction brings us closer to solving the problem. Like the movement “pick up one garbage a day” started with a few people and spread wide and far, so can the movement “stop antimicrobial resistance” be equally powerful if enough people take part in and support it.
further reading & resources
“Antimicrobial resistance (AMR) – World Bank”
“ND4BB – New Drugs for Bad Bugs – IMI”
“GUARD Follow-up Report – Federal Ministry of Health”
“Big Pharma’s New Antibiotics Are Failing as Infections Grow Stronger”
“Why are there so few antibiotics under development?”
“The Race to Save Antibiotics”
“Antibiotic / Antimicrobial Resistance (AR / AMR) | CDC” –
references
Chattopadhyay, M.K. (2014). Use of antibiotics as feed additives: a burning question. Front Microbiol, 5:334. doi: 10.3389/fmicb.2014.00334.
Clatworthy, A.E., Pierson, E., Hung, D.T. (2007). Targeting virulence: a new paradigm for antimicrobial therapy. Nat Chem Biol, 3(9):541-8. doi: 10.1038/nchembio.2007.24. PMID: 17710100.
Koch, N., Islam, N.F., Sonowal, S., Prasad, R., Sarma, H. (2021). Environmental antibiotics and resistance genes as emerging contaminants: Methods of detection and bioremediation. Curr Res Microb Sci, 2:100027. doi: 10.1016/j.crmicr.2021.100027.
Kraker De MEA, Stewardson AJ, Harbarth S. (2016). Will 10 Million People Die a Year due to Antimicrobial Resistance by 2050? PLOS Medicine, 13:1-6. DOI: 10.1371/journal.pmed.1002184
Landers, T.F., Cohen, B., Wittum, T.E., Larson, E.L. (2012). A review of antibiotic use in food animals: perspective, policy, and potential. Public Health Rep, 127(1):4-22. doi: 10.1177/003335491212700103. PMID: 22298919; PMCID: PMC3234384.
Llor, C., Bjerrum, L. (2014). Antimicrobial resistance: risk associated with antibiotic overuse and initiatives to reduce the problem. Ther Adv Drug Saf, 5(6):229-41. doi: 10.1177/2042098614554919.
Nelson, R. (2003). Antibiotic development pipeline runs dry. Lancet, 362(9397):1726-7. doi: 10.1016/s0140-6736(03)14885-4. PMID: 14655659; PMCID: PMC7135628.
O’Neill, J. (2016). Tackling drug-resistant infections globally: Final report and recommendations. London: HM Government and Wellcome Trust; Review on Antimicrobial Resistance, chaired by Jim O’Neill.
Polianciuc, S.I., Gurzău, A.E., Kiss, B., Ştefan, M.G., Loghin, F. (2020). Antibiotics in the environment: causes and consequences. Med Pharm Rep, 93(3):231-240. doi: 10.15386/mpr-1742. Epub 2020 Jul 22. PMID: 32832887; PMCID: PMC7418837.
Silver, L.L. (2011). Challenges of antibacterial discovery. Clin Microbiol Rev, 24(1):71-109. doi: 10.1128/CMR.00030-10. PMID: 21233508; PMCID: PMC3021209.
Terreni, M., Taccani, M., Pregnolato, M. (2021). New Antibiotics for Multidrug-Resistant Bacterial Strains: Latest Research Developments and Future Perspectives. Molecules, 26(9):2671. doi: 10.3390/molecules26092671.